A Case for Curiosity
By Robert Levy
In a pragmatic, goal-oriented age, the notion of curiosity as the driving force behind research might seem out of fashion. But in the long run, it may be curiosity — unimpeded and open-minded — that cures cancer.
For evidence, one need look no further than the 2019 Nobel Prize in Physiology or Medicine, awarded to Dana-Farber's William Kaelin Jr., MD, Sir Peter Ratcliffe of the University of Oxford, and Gregg Semenza, MD, PhD, of Johns Hopkins University.
At the outset, it was far from obvious that Kaelin's work would contribute to a pivotal discovery about how cells adapt to changes in oxygen levels. But because it sprang from a desire to answer a fundamental biological question, and a willingness to follow a course of research wherever it led, it would be unwise to bet against its potential.
Long before the morning he learned he had co-won medicine's highest award, Kaelin, the Sidney Farber Professor of Medicine at Dana-Farber and Harvard Medical School, senior physician in Medicine at Brigham and Women's Hospital, and Howard Hughes Medical Institute investigator, has been making the case for curiosity-driven research and its potential to transform the treatment of disease.
"The beauty, as well as the challenge, of basic science is that you can't always predict what the outcome of the work is going to be," he says. "You're investing in the creation of new knowledge, and the only thing you can be sure of is that the more you invest in basic science, the more new knowledge you will generate. And the more knowledge we have, hopefully, the more improvement we will have in the quality of our lives."
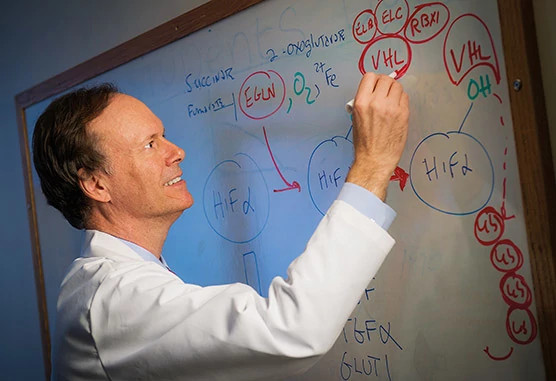
The Right Spot
Choosing an area of research is, Kaelin likes to say, like fishing: It's ultimately guesswork, but guesswork informed by experience and a sense of where the greatest promise lies. As a budding laboratory scientist in the early 1990s, he was looking for something that was interesting not only in itself but as a source of clues into a variety of cancers — something where research could be informative as well as impactful.
The answer came in 1993 when he read a report in Science magazine about the cloning of a gene called VHL. "The moment I saw the paper I said, this is perfect, this is what I should work on," Kaelin relates.
The gene met Kaelin's criteria for a subject at the hub of multiple questions in cancer. People with a mutated, or abnormal, VHL gene are at high risk of developing a rare disorder called von Hippel-Lindau (VHL) syndrome in which tumors and cysts form in multiple organs and tissues.
Although some of these growths are benign, they can be harmful if they press on or interfere with neighboring tissues. Some of the tumors, such as kidney cancers, are malignant and can spread to other parts of the body. Research into the disorder offered a way to help patients and, Kaelin intuited, generate broader insights. Scientists quickly determined that the VHL gene also plays a critical role in non-hereditary kidney cancer.
Kaelin thought that studying the VHL gene might provide important insights into the common form of cancer.
Because tumors associated with VHL disease are often rich in blood vessels, studying them could also help explain how tumors reroute the bloodstream for nourishment. And because people with VHL tumors can develop a sharp increase in red blood cell production – normally a sign that tissues aren't getting enough oxygen — VHL research could shed light on how cells sense and respond to changes in oxygen levels.
It was the last of these possibilities that would yield discoveries deserving of a Nobel Prize.
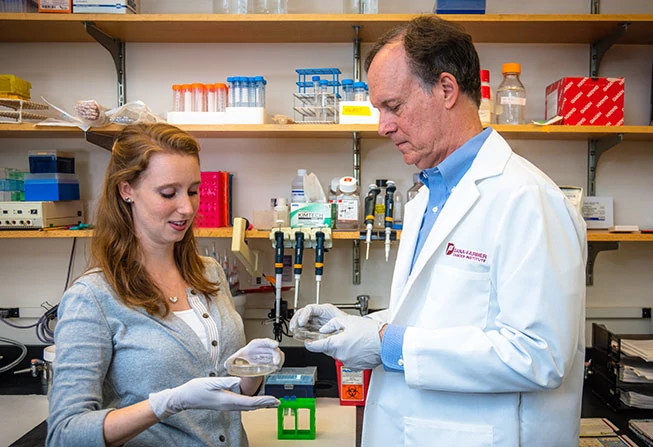
A Monopoly on Oxygen
At first, it might seem that questions about cells' capacity for adjusting to changes in oxygen have more to do with mountain climbing than malignancy. In virtually all animals, oxygen is needed to convert food energy into a useful form.
When the demand for oxygen exceeds the available supply — at high elevations, for example, or during exercise — the body responds by ramping up production of red blood cells (which carry oxygen by binding it to hemoglobin), building new blood vessels (to channel more blood cells to tissue), and activating glycolysis (a series of reactions that extract energy from blood sugar).
A variety of diseases can prompt the body to take these steps, known as the hypoxia response, even though environmental levels of oxygen are normal — stroke, heart disease, anemia, infection, and, perhaps surprisingly, cancer.
Cancer cells are hoarders at the bloodstream's banquet of nutrients. To fuel their breakneck-growth, they demand an extra helping of oxygen. To get it, they send out distress signals that spur the formation of red blood cells and blood vessels. Understanding how cancer cells hijack the hypoxia response would be a first step toward blocking it — and potentially starving the cells of a fuel they need to survive.
By the late 1990s, several lines of research were zeroing in on cells' machinery for gauging changes in oxygen levels and adjusting to them. Kaelin's group had theorized that the VHL gene was involved in oxygen sensing: the fact that VHL tumors are laced with blood vessels and laden with red blood cells suggested that when the gene is mutated, the sensing mechanism is broken.
To test the theory, they created human kidney cancer cell lines that were identical except that one had a normal version of the VHL gene and the other didn't. They grew them in either a high- or low-oxygen environment and looked for the presence of RNAs that normally are produced only when oxygen levels are low.
"My jaw dropped when I saw the results," Kaelin recalls. "We found that cells without the normal VHL gene overproduced those RNAs whether oxygen was present or not. It confirmed that the gene was required for oxygen-sensing."
This finding, from 1996, was a major advance, but researchers didn't know precisely how changes in oxygen flipped this molecular switch. Semenza had found that when oxygen levels are high, a protein complex called HIF-1 is steadily eroded, but when they're low, HIF-1α builds up. The discovery quickly converged with Kaelin's work. In 2001, Kaelin and Ratcliffe independently showed that under normal oxygen conditions, cells place a chemical tag on HIF-1, prompting the VHL protein to destroy it. When VHL is missing or deformed because of a genetic mutation, HIF-1α doesn't get degraded, causing the cell to act as though oxygen levels are low.
The sense of curiosity that drove the researchers had brought them to a molecular mechanism that was itself a rather curious bit of cellular operations. The HIF-1α complex functions, in a way, like a reverse thermometer: when oxygen levels are adequate, HIF-1α recedes; when the levels drop, HIF-1α rises, ordering delivery of a kind of oxygen mask to cells gasping for air.
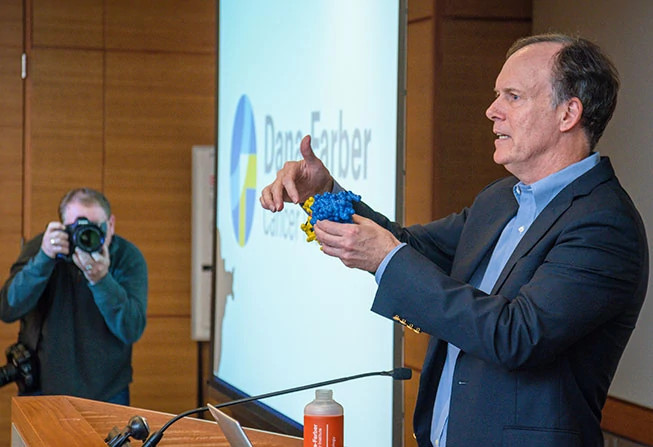
Coming to the Clinic
Following the 2001 breakthrough, Kaelin, Semenza, and Ratcliffe uncovered more details of the oxygen-response system and showed that malfunctions in the system can play a role in cancer and other diseases. Kaelin, for example, found that a defect in HIF-2α — a close kin of HIF-1α — propels the growth of certain kidney cancers and that of triple-negative breast cancers often make use of HIF-1α.
Researchers are also working on practical applications of their discoveries. "Now that we've mapped the molecular pathway behind the hypoxia response, we have clues about where to intervene to make the pathway more or less active," Kaelin remarks.
"For example, we now have drugs that prevent HIF-1α from deteriorating, which might be useful for diseases like anemia, heart attack, and stroke, where oxygen delivery is problem. Conversely, blocking the pathway, with HIF-2α inhibitors, for example, looks like a promising way to treat kidney cancer. I'm also hopeful that HIF-1α inhibitors might be useful for certain cancers."
The Nobel Prize win has amplified Kaelin's challenge to those who believe curiosity-based research is too unreliable to undertake or invest in. From experience, Kaelin knows that science doesn't exist to satisfy curiosity but to perpetuate it – that discoveries are not goals as much as they are incentives to pursue more research. At the most basic level, curiosity-driven research is an expression of confidence in the mind's ability to make connections, perceive promise, and inspire others.
"Some people might think it risky to have talented scientists follow only their curiosity, because we won't necessarily be able to predict where the road will take them and we won't be able to predict what the fruits of their labors will be," Kaelin asserts. "But we know, over and over, that's how real progress happens."
Request a Publication
Receive by mail the current issue of a Dana-Farber publication by completing this request form.
Media Contacts
If you are a journalist and have a question about any of our stories or need more information, email media@dfci.harvard.edu or call 617-632-4090 and ask to speak to a member of the media team.
The Media Team cannot respond to patient inquiries. For more information on contacting Dana-Farber, please see Contact Us.
Disclosure of Advisory Roles and Certain Industry Support
See an overview of relevant board memberships, outside support, and significant advisory roles as reported by several physicians and researchers featured in the 2020 issue of Dana-Farber's Paths of Progress magazine.
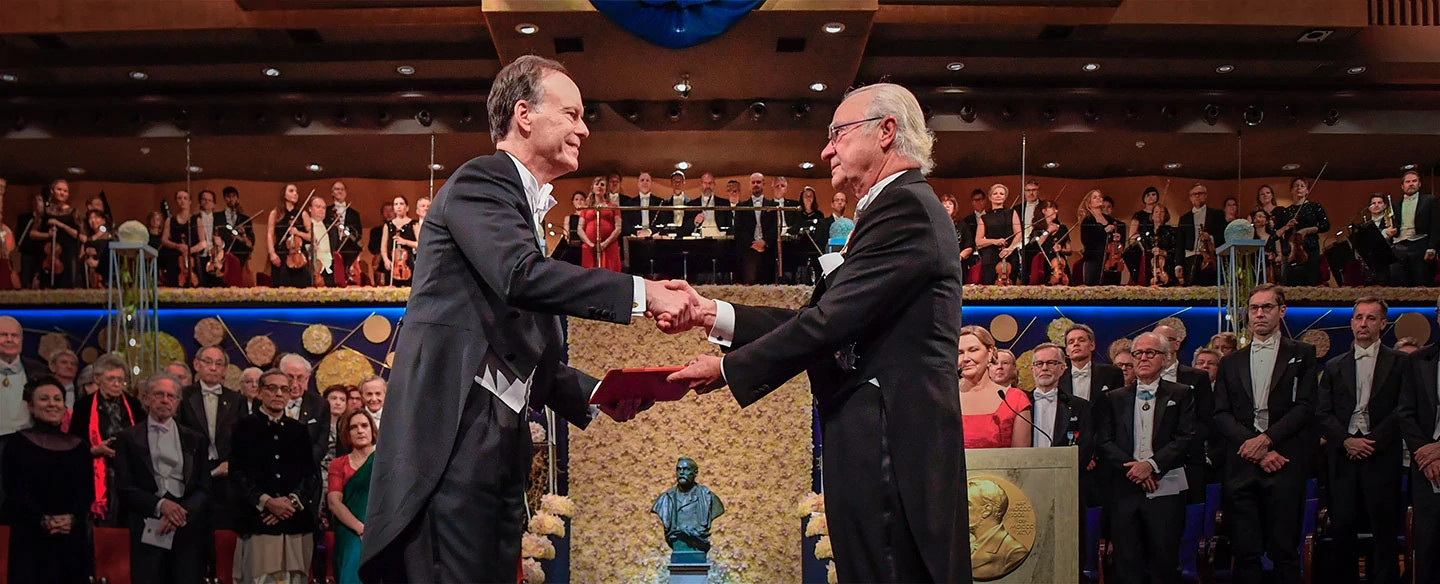

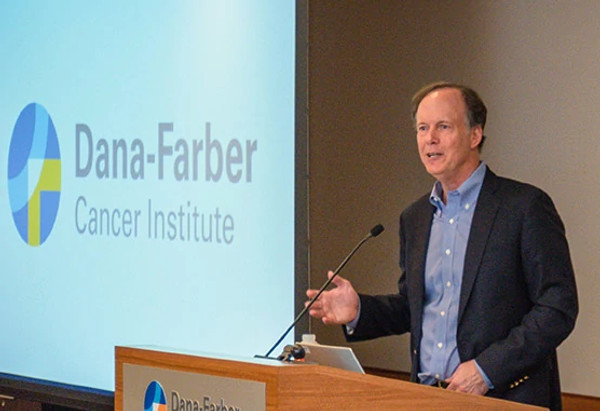
William G. Kaelin Jr., MD, Wins 2019 Nobel Prize in Medicine
Read about Dr. Kaelin's award, his scientific discoveries, his reactions to winning the award, and much more.
Other Feature Stories from Paths of Progress 2020
-
Unexpected Benefits from Targeted Therapies
New research shows that CDK 4/6 and PARP Inhibitors can stimulate the immune system to fight cancer, in addition to their known effects in fighting tumor cells.
-
Shapeshifters in the Brain
Understanding how aggressive brain tumors evade treatment with new technology that examines genetic information within individual cells, one at a time.
-
Bringing AI to Personalized Cancer Care
Machine language technology brings the new capability to study each individual's risks and best treatment options.
-
Liquid Biopsies Move Toward the Clinic
Chemical biologists create small molecules to boost discoveries and prototype novel therapies.
To see the entire publication, please download the PDF version.